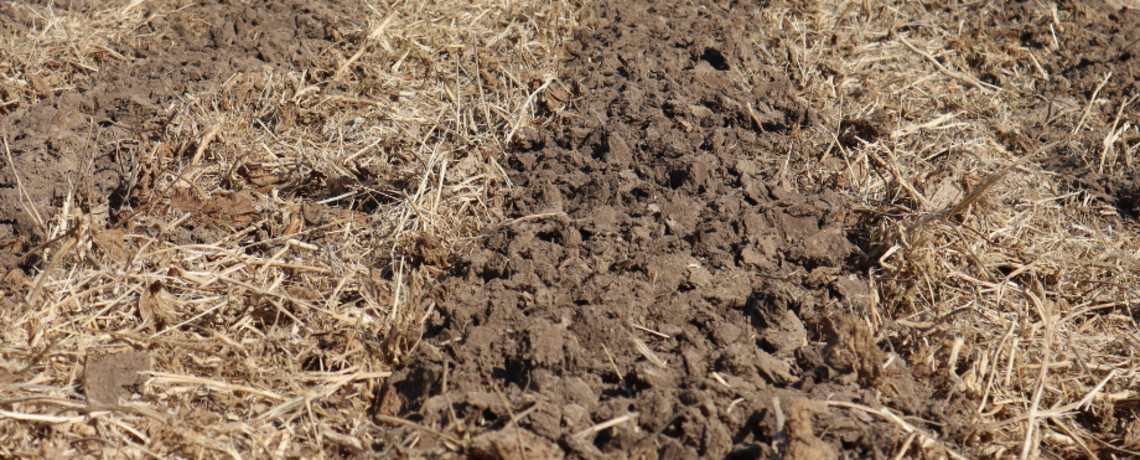
Soil Organic Matter: Its Functions and Value
Written by: ForGround by Bayer
Summary:
- The soil organic matter pool is a large source of the world’s carbon
- The cycling of organic matter is a continuous and dynamic process and additions of new organic residues are required to maintain organic matter levels
- Different fractions of organic matter behave differently in what they do and how quickly they can rise or fall
- Small changes in the percent organic matter can greatly affect soil properties
- Increasing soil organic matter generally has positive impacts on soil fertility, water movement and other soil properties.
Read time: 8 minutes
Organic matter is a driver of many important soil processes and many farmers are managing their farms with the goal of increasing organic matter. While most recognize that organic matter is a key driver of a healthy soil, how it is formed and its value is much more nuanced. Therefore, an understanding of its function is important in managing soils to their fullest potential.
Our World’s Supply of Soil Organic Carbon
The total amount of carbon stored in the world’s soils is estimated at approximately 2.5 trillion metric tons, twice that present in the earth’s vegetation and atmosphere combined (Lal, 2004). The carbon storage abilities of the world’s soil is a double-edged sword; an estimate of soil carbon lost since 1850 is 78 billion metric tons, and on average the world’s soils are continuing to lose carbon (Lal, 2007). However, the potential storage capacity of the world’s soils is estimated to be as high as 52 billion metric tons, meaning that there is great opportunity to sequester atmospheric carbon with our soils.
Soils are continuously turning over organic matter, resulting in the release of nutrients and carbon dioxide. This release of carbon dioxide is not inherently bad, as carbon dioxide is a necessary input for photosynthesizing plants. And when soils are left in an un-disturbed state, the amount of carbon dioxide liberated by soils typically achieves a steady state with the amount sequestered through the addition of organic matter. It is through the disturbance of soils that the release of carbon can exceed the gains therein.
The rate at which soils gain or lose organic matter is affected by climatic conditions on both global and local scales. In each scenario, there two factors at work; those influencing the growth of plants (organic matter addition) and those influencing organic matter retention. If an area can produce plant residues faster than it can be broken down, organic matter will increase. Globally, soil organic matter accumulates fastest in cool, wet environments and is lost the quickest in hot, dry environments. One can easily visualize this when thinking of the deserts of the southwest United States vs the Northern Great Plains in eastern North Dakota or Minnesota. On a more local scale, the same effect applies. Soils that tend to be drier, shallower and have lower productivity (the tops of hills for instance) tend to accumulate organic matter slower than those in lower landscape positions that are wetter, deeper and support greater plant production.
Organic Matter Composition and Processes
Soil organic matter is comprised of many different parts that we can roughly place into four different categories: living microorganisms, fresh residue, active (quickly degrading) carbon sources and passive (slowly degrading) carbon sources. The active and passive fractions typically comprise 1/3 to ½ of a soils total organic matter pool. The passive fraction consists of slowly degrading materials such as humic substances that are extremely stable and may remain in a soil for hundreds or thousands of years. These substances account for much of the organic matter influence on cation exchange capacity, or the ability to hold on to nutrients in the soil solution, and soil water retention capacity. Passive organic matter also includes slowly degradable material, including the fiber tissues of cellulose and lignin, along with fats, waxes and phenolic compounds that may take decades to break down and these substances can account for much of the mineralizable nitrogen in a soil
At the other end of the organic matter spectrum, there is the quickly decomposing active fraction. Active organic matter is comprised of easily decomposable plant root exudates and acids and decaying simple organisms, such as bacteria, and may last from months to a few years. This fraction includes glomalin, an exudate produced by mycorrhizal fungi that cements soil particles together forming aggregates, which ultimately affect many of the positive soil properties associated with soil health (Comis, 2002). Materials like and including glomalin are some of our least understood and historically underestimated components of organic matter; it has only been within the past few decades that scientists have identified and began to study these materials.
The short-term nature of active organic matter means that it is highly responsive to changes in land management. Because active organic matter is only a slice of the total organic matter pie, yet it is responsible for so much function, increasing the size of that slice, which may not increase total organic matter by a large amount, can have big effects on soil health and function. This also means that one needs to continuously add and/or prevent the loss of this quickly eroding pool of material.
The loss of organic matter in agricultural systems is typically an oxidation process, which is often accelerated through tillage. When compared to natural soil processes, tillage is a fast, violent event that rapidly changes soil physical and biological properties. First, the tillage process physically breaks down soil aggregates and destroys pore networks, reducing water flow and retention and making soils more susceptible to compaction. Second, tillage disrupts beneficial soil organisms such as earthworms and beneficial fungi. Finally, the rapid aeration of the soil speeds up the microbial processes that consume organic matter, rapidly converting soil carbon into carbon dioxide. Therefore, one of the most straightforward means of stemming the loss of soil carbon is to reduce or eliminate tillage.
While tillage slows the loss of soil carbon, it does not necessarily add more to a soil system. This is primarily accomplished by adding organic feedstocks for soil microbes to covert to organic matter. This includes physical additions, such as manure, but also the additions of above and below-ground plant residues. In managing cash crops, this means maximizing plant growth through optimum fertility, although excessive fertility can have the reverse effects as it can speed up the process of organic matter oxidation. This also achieved by providing actively growing plant roots throughout the year, typically through cover crops. For instance, over 3,000 lbs. of root mass per acres can be added to a soil through a cover crop in a single year (Sievers, 2018). What is important to remember is that if you’re not adding organic matter, you’re losing it, as the natural process of oxidation slowly chips away at organic matter reserves.
What is the Value of Organic Matter?
While organic matter typically comprises a small amount of the soil (typically 2-5%), it drives many critical soil properties and functions. While some benefits of increased organic matter, such as increased nitrogen are more objective, other aspects, such as increased compaction resistance tend to be more subjective. When determining the value of organic matter, it may be best weigh both aspects, as it affects farm productivity and the broader agricultural ecosystem in many different ways.
Soil Macronutrient Contributions
In a 2-million-pound acre furrow slice of soil, each 1% of organic matter equates to 20,000 lbs. of material. A soil will turn over approximately 2% of its organic matter annually, so for every 1% of organic matter, approximately 400 lbs. of organic matter is cycled annually. Soil organic matter contains nitrogen, phosphorus, sulfur, and other trace elements. In a healthy functioning soil, the total amounts released will vary based on soil conditions, with a general range noted in table 1.
Table 1: Annual nutrient release of nutrients (in lbs. per acre) at 1-5% soil organic matter.
As the table shows, as organic matter climbs the amount of available nitrogen can be rather meaningful in a farm’s nutrient management plan. While the nitrogen release from organic matter oxidation is more of a “slow burn,” release picks up as temperatures warm, often coinciding with the summer crop growth cycle. Depending on specific crop fertility timing needs, organic matter contributions may not replace supplemental fertilizer one-for-one, but when used with adaptive nutrient management practices such as nitrogen estimation tools, pre-sidedress testing or chlorophyll meter monitoring, sizable reductions in applied nitrogen can be had.
Micronutrient Chelation and Plant Growth Stimulation
In addition to the nutrients released by organic matter, the presence itself of organic matter can affect plant production. Humic acids can stimulate plant growth and root development, in some plants including corn and soybeans (Tan, 1979; Tan, 1983). Humic acids also chelate, or bind nutrients such as iron, copper, zinc and manganese into stable complexes that more easily accessed by plants.
Increase in CEC and buffering of pH
As organic matter takes on a more stabilized form, it gains an ability to hold onto plant nutrients, increasing the cation exchange capacity (CEC) of a soil. This is especially important in sandier soils, with low inherent CEC, where nutrients are quickly leached. An increase of CEC in those soils can ultimately result in more plant-available nutrients, greater flexibility in nutrient application or fewer nutrients applied. Organic matter also binds hydrogen ions, ultimately buffering against the acidification of soil.
Improved Soil-Water Properties
Soil-water processes often start with water droplets contacting the soil typically through rainfall or irrigation. Although we often perceive rainfall and irrigation as a gentle process, this can be a violent action at microscopic scale, with a tiny explosion from each raindrop detaching soil particles and initiating the process of soil erosion. Exposed surfaces are also prone to crust formation, which greatly impacts infiltration rates, even when the soil below is well aggregated with lots of macropores. Surface residues absorb the impact of precipitation and the labyrinth of residue that a water droplet follows to reach the soil surface attenuates quick bursts of rainfall, ultimately making a field less “flashy” and allowing more water to infiltrate.
Once water enters the soil profile, soil pores begin to fill. However, if those pores are too small and poorly connected, they may remain filled for too long and oxygen becomes limited, stunting plant growth and allowing anaerobic bacteria to convert soil nitrate to nitrogen gas through the process of denitrification. Additionally, smaller soil pores hold onto water so tightly that plants cannot access it, even though it may be present in the soil. Organic matter and the practices that increase soil organic matter, such as reduced/no-tillage and cover crops, promote the formation of macropores that hold soil water yet drain quickly enough to allow oxygen to enter the soil profile. Finally, the sponge-like nature of organic matter help to retain and provide more plant-available water, as its ability to hold soil water is much higher than that of the mineral particles that comprise the bulk soil.
What does this mean in the context of water management? First, organic matter generally increases the total infiltration from a rainfall or irrigation event, through the ability to infiltrate quick bursts of rainfall and the ability to infiltrate more across a storm event. Second, organic matter aids in the aggregation processes that create larger pores that hold more water and allow for more water to be available to plants. Finally, those same pores drain easier, returning soils more quickly to the “ideal” balance of air and water necessary for optimum root and plant growth.
Compaction Resistance
The aggregation of particles with the help of organic compounds, such as glomalin, also aids in resisting soil compaction. As greater amounts of soil glue are exuded by arbuscular mycorrhizal fungi, aggregates are strengthened and are less likely to rupture under the forces of compaction. This ultimately results in improved plant growth and reduced rutting in the field or the ability to perform fieldwork sooner while still maintaining a desirable level of tilth suitable for no-till and germinating seedlings.
What Does it all Mean?
While placing a value on organic matter can be subjective at times, any action to increase it will ultimately bring a benefit. Values of fertility will fluctuate with fertilizer prices and the value of additional water or resilience to extreme weather events will likely vary given the year. However, the outcomes, such as needing to irrigate less, or increased yield due to lower plant moisture stress are certainly real and often consistent. Additionally, the soil structural effects are real, and their savings may be as straightforward as additional yield due to better root growth due or more nuanced, such as in the savings of fuel, equipment hours and manpower necessary to mechanically remediate compaction. It’s ultimately up to each individual farmer to determine how much this is all worth, but for those that see the big picture of soil carbon, there is great value in trying to build more year after year.
Sources Cited:
Brady. N. C. & Weil R. R. (2002). The nature and properties of soils (13th Ed.). Prentice Hall. Comis, D. (2002, September). Glomalin: Hiding place for a third of the world’s stored soil carbon. Agricultural Research, 4-7. Lal, R. (2004). Soil carbon sequestration impacts on global climate change and food security. Science, 304, 1623-1627. Lal, R., Follett, R. F., Stewart, B. A. & Kimble, J. M. (2007). Soil carbon sequestration to mitigate climate change and advance food security. Soil Science, 172(12), 943-956. Doi:10.1097/ss.0b0131815cc498. Sievers, T., & Cook, R. L. (2018). Aboveground and root decomposition of cereal rye and hairy vetch cover crops. Soil Science Society of America Journal, 82, 147-155. Doi: 10.2136/ssaj2017.05.0139. Tan, K. H. & Nopamornbodi. V. (1979). Effect of different levels of humic acids on nutrient content and growth of corn (zea mays L.). Plant and Soil Science, 51, 283-287. Tan, K. H. & Tantiwiramanond, D. (1983). Effect of humic acids on nodulation and dry matter production of soybean, peanut and clover. Soi Science Society of America Journal, 47, 1121-1124.
Legal Statement:
ALWAYS READ AND FOLLOW PESTICIDE LABEL DIRECTIONS. Performance may vary, from location to location and from year to year, as local growing, soil and weather conditions may vary. Growers should evaluate data from multiple locations and years whenever possible and should consider the impacts of these conditions on the grower’s fields.
Bayer and Bayer Cross are registered trademarks of Bayer Group. All other trademarks are the property of their respective owners. ©2023 Bayer Group. All rights reserved.